Advancing Critical Infrastructure
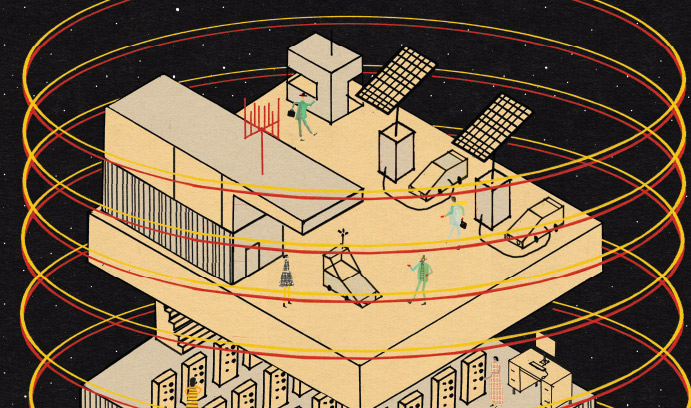
The countless man-made physical systems that keep us safe, comfortable and connected—buildings, roads, bridges, tunnels, transportation systems, the energy grid, water and sanitation systems, the Internet—are integral to modern life. And although they might go unnoticed or unappreciated in the day-to-day, we certainly notice them when something goes wrong: a massive power outage, a train derailment, a bridge collapse.
In 2017 alone, severe weather events including Hurricanes Harvey, Irma and Maria pushed the infrastructure in those impacted areas to the very brink of—and sometimes beyond—their limits, often with dire consequences. And as infrastructure ages, the challenges and risks only grow more significant.
Lehigh researchers have long sought to meet infrastructure challenges. With an eye toward the interdependencies between these indispensable systems, researchers are working on a wide range of research that spans various fields of study to develop sustainable and resilient infrastructure and energy systems. Lehigh in April launched its new Institute for Cyber Physical Infrastructure and Energy, one of three inaugural Interdisciplinary Research Institutes (see sidebar), which will further strengthen and support this work.
Cyberphysical Systems for Improved Performance
In 2017, America’s infrastructure received a grade of D+ by the American Society of Civil Engineers, which issues a report card every four years.
However, the communication infrastructure tends to be most up-to-date, says Shalinee Kishore, Iacocca Chair and professor of electrical and computer engineering.
“[It] can be used to help some of the other infrastructure systems work a little better, because in the end, those infrastructure systems are now going to be cyber-physical systems,” she says.
A “cyber-physical” system is, generally speaking, a physical system with cyber components that take measurements, gather information, and allow for automated decisions and changes in the physical system. The application of digital communications technology to any physical system—the U.S. power grid, a manufacturing facility, a city’s transportation system, a bridge—is relatively cheap. Operators of these infrastructure systems use data collected from a variety of sources to make decisions about how to control the physical system or to determine what adjustments the system might require. Whether it’s gathered from sensors physically attached to a structure, smartphones carried by users or customer comments posted on social media, the data can help develop a clearer understanding of how a system is functioning. This is particularly useful with aging infrastructure, says Kishore.
“The idea is to use the data to make all those aging infrastructure systems work better, to figure out what to upgrade, how to upgrade, how to make it work with the retrograded parts of the system,” says Kishore. “And then infrastructure systems themselves are interrelated, so how you use the energy infrastructure and how you use the transportation infrastructure is very correlated. How does that data that you're learning about one system versus the other tell you about both systems at once, and how could you make them jointly work better?”
The key question, says Shamim Pakzad, associate professor of civil and environmental engineering, is: “How can we use data to improve the quality of life in urban settings within the constraints that we have in the U.S. and in other industrial communities?”
Monitoring Bridge Health
Pakzad uses data to assess the safety of the bridges that connect communities.
Ten years ago, while working toward his Ph.D. at UC Berkeley, Pakzad used fixed sensors to monitor the conditions of San Francisco’s famed Golden Gate Bridge.
“For our project, we spent $25,000 to attach 320 sensors to the bridge, creating a wireless sensor network,” says Pakzad. “Over a more than three-month deployment period, the amount of data we were able to collect was, for that time, unbelievable.”
As impressive as that was, the volume of data generated by the millions of connected mobile devices crossing the Golden Gate bridge—inside the 100,000 vehicles, on average, that cross daily—dwarfs the amount of data that any fixed sensor network can obtain, either 10 years ago or today.
“How can we not use that data?” asks Pakzad. “It may not have the same quality as the dedicated, fixed sensor networks, but it still has value, and we want to extract all of the juice from it.”
How to extract the juice—or meaningful information to analyze—is the subject of Pakzad’s National Science Foundation (NSF) Faculty Early Career Development (CAREER) Program award, which supports Pakzad’s goal to establish a long-term research and educational program focused on the application of mobile sensors to assess bridge conditions.
Data related to acceleration and vibrations are of particular value, as it could be used to measure structural response—such as the condition of the pavement and the presence of potholes—as well as environmental conditions at rates, volumes and locations not possible in the past. One of the reasons that the current U.S. bridge conditions monitoring system is problematic, according to Pakzad, is that bridges are only required to be inspected every two years. A lot can happen between these mandated visual inspections, he says.
“The current system is also limited to what can be seen,” says Pakzad. “We are trying to develop methods to inform us about areas within the structure that are not visible, but are already impacting the performance and condition of the structure.”
To fully leverage big data for structural health monitoring, new analytical tools must be created. According to Pakzad, these tools must be adaptable, in order to process data from different sensing networks, as well as computationally scalable, to handle any number of sensors and sensing nodes represented by a given data set.
Pakzad and his former student, who is now at the Massachusetts Institute of Technology, proposed a method, called STRIDEX, that is the first output-only modal identification (a way of identifying certain properties of a structure based on vibrational data) to be both adaptable and scalable. In experimental results using the STRIDEX algorithm, a mobile sensor was shown to provide over 120 times more mode shape points—how vibration characteristics are measured—than a fixed sensor. These results were published in Computer-Aided Civil and Infrastructure Engineering earlier this year.
“The ability to extract detailed structural system information from a mobile data network in a computationally scalable framework is a key step toward being able to use mobile sensing data to monitor structures in a large urban setting,” says Pakzad.
Pakzad is also working with Martin Takáč, assistant professor of industrial and systems engineering, on applying deep learning techniques to structural health monitoring. A paper they co-authored, “Innovative Sensing by Using Deep Learning Framework,” was presented at a premier conference in modal identification and damage detection, and will be published later this year in Dynamics of Civil Structures.
Watts, Bits and Dollars
Within the U.S. power grid lie three interdependent networks: power, communication and money—or, as the members of Lehigh’s Integrated Network for Electricity (INE) research cluster like to say, watts, bits and dollars.
Lehigh in 2011 established the INE cluster, which includes faculty from a variety of disciplines focused on examining the electricity grid as an integrated “network of networks.” Since its inception, the group has produced significant research in the areas of cybersecurity for the electrical power grid as well as energy management systems, power system reliability, renewable integration, and the development of ocean wave energy and other marine hydrokinetic energy systems. Nine cluster members are among the recipients of a $12.2 million multi-university grant from the U.S. Department of Energy to develop an interdisciplinary approach to integratable, composable and evolvable cybersecurity in energy delivery systems. Two separate National Science Foundation grants have been awarded to teams of researchers to study ocean wave energy.
Kishore, along with Rick Blum, the Robert W. Wieseman Professor of Electrical Engineering, and Alberto Lamadrid, an assistant professor with a joint appointment in economics and industrial and systems engineering, are investigators on the DOE grant and are among the large group of Lehigh faculty working to make the U.S. power grid more sustainable and resilient.
“Everything depends on the grid,” says Blum. “And then, if you start thinking about it, the grid actually depends on other things as well. We need all of the various generation sources to supply this electrical energy to the grid.
The grid network runs on a simple, automated principle: Energy is generated based on demand. When more demand is anticipated, generators can burn more coal or fuel, for example, to match that demand.
However, aging equipment, changing regulations and the addition of renewable generation such as wind and solar energy have added complexities put strain on a system that has been mostly reliable over the years, Kishore says. Over the past few decades, deregulation at the wholesale and market levels has moved the energy industry away from the highly structured, vertically integrated monopolies that once dominated electricity production. Customers now have more options and, thanks to the communication network atop the grid network, in the near future will be more aware of and reactive to current conditions.
Just as power flow conditions influence the communication between the power company and its customers, the type of communication shared can determine power flow. When customers have access to information about high-price times and low-price times, demand becomes more responsive. Customers can respond to prices as they make decisions about their energy consumption. The three networks—watts, bits and dollars—work together, connected and dependent upon one another.
Exploring Lesser-Known Interdependencies
“There is very small latency in the electricity system. Supply and demand have to be balanced in very short time scales, and there’s very little storage,” says Lamadrid. “So, what happens the moment that you start having more variable and uncertain energy?”
Many forms of renewable generation cannot be predicted easily—wind and solar generation, for example, depend upon weather conditions. This dependency makes wind and solar a volatile supply, requiring a form of backup generation to meet customer demand. In many parts of the United States, that backup is natural gas: a clean, affordable, widely available resource. The INE team is now examining the interaction between the electrical and natural gas networks.
“The problem is that the two systems are coupled, but we don’t fully understand or manage the coupling. In the current way that we manage the electrical grid, we essentially ignore the natural gas network. We want to try to somehow take both networks into account during the planning of their operation,” says Blum.
In addition to electricity generation, natural gas is also used for heating in colder climates. As priority is given to heating customers, natural gas can become an unreliable resource for the power grid.
“If you’re in a place like New England, it’s probably more important to warm up your house than using natural gas in order to produce electricity,” says Lamadrid. “So, you end up in this situation in which you have very constrained pipeline capacity for natural gas, and therefore the system operator, which is already very reliant on that natural gas, may not have any fuel to start powering the system. … It doesn’t happen very often, but you may end up with either very high prices or some curtailments. You’re going to be not serving some of this load because economically we cannot actually fulfill it.”
This affects the markets, says Lamadrid. Despite their interdependency, the two systems are administered very differently, and each has its own incentives. The Lehigh team is interested in how to coordinate the markets across the two systems to reduce risk and vulnerability.
“There are some aspects of game theory here because you can just think that in the case of the natural gas, there may be some producers that want to maximize their profit, get as much money as possible from that production. And on the other hand, you have the system operator, whose interest is not in maximizing money but is on maximizing welfare. So, we’re going to be having something that [the first] entity is going to be making a decision, [and the second] entity is going to be making the decision after they observe what the first one did. And then there is going to be some interaction between the two of them,” says Lamadrid.
Potentially Dangerous Connections
The benefits of web-based interactions between systems are accompanied by some risk. Individuals who seek to exploit the interconnection between systems can impact the grid, as well as any other systems dependent upon it.
“We can imagine hazards to infrastructure from things like hurricanes and earthquakes, terrorist attacks—those are easily imagined,” says Richard Sause, the Joseph T. Stuart Professor of Civil and Environmental Engineering and director of the ATLSS Engineering Research Center. “But I think we’re getting to the point where an attack by hackers that’s coordinated and well-planned can do essentially the same thing. So, it’s a hazard of a different kind, and we need to think about how to defend against that hazard. The whole community is at risk in some way.”
For example, in the case of the power grid and natural gas, “if someone disrupts the natural gas sources or delivery network, that’s going to affect the electrical grid since some generators in the grid run on natural gas. And the electrical grid itself provides electrical energy that’s used to produce and transport the natural gas to the places that need it. So, if the electrical grid goes down, that would also disrupt the natural gas network. These interdependencies can cause some serious problems,” says Blum.
Solving problems like these requires researchers with expertise in a wide range of topics: the grid itself, the grid’s communications network, encryption and decryption, and privacy. Members of the INE research cluster have published and presented numerous papers on cybersecurity for the smart grid. Among them is a 2017 paper for the Conference on Information Science and Systems titled “Network Topology Risk Assessment Of Stealthy Cyber Attack On Advanced Metering Infrastructure Network” by Kishore; Blum; Larry Snyder, associate professor of industrial and systems engineering; Parv Venkitasubramaniam, associate professor of electrical and computer engineering; and Jiyun Yao, then a Lehigh doctoral candidate in electrical engineering.
Lehigh researchers work on creating models to determine how an attacker might misuse, for example, the demand-side management component of the smart grid to benefit financially or to simply wreak havoc, as well as what the grid operator can do to detect or mitigate such an attack.
Snyder, whose background is in supply chains under the risk of disruption, provides the mathematical optimization models that advance this type of work.
“If we want to have a good model of the worst a bad guy could do to the system, that’s an optimization problem,” he explains. “How does a bad guy maximize the damage he can cause if he has limited resources to perpetrate the attack? And the flip side of what the power company can do to mitigate that is also an optimization problem: We have only so much money available. How can we best protect the grid against these attacks, given that the bad guys are going to do their worst?”
Managing Risk from Natural Disasters and Climate Change
Cyber-attacks aren’t the only threats to our collective infrastructure systems. Natural disasters such as floods and hurricanes can have devastating effects. Lehigh researchers tackle these problems as well.
During a natural disaster, connecting people by bridges can mean the difference between life and death. And, in the aftermath of such extreme weather events, bridges are also crucial for recovery efforts. Dan Frangopol and his research team use probabilistic modeling and analysis, as well as advanced computer simulation, to assess the effects of natural disasters and other uncertainties on infrastructure.
In their latest research, Frangopol, the inaugural Fazlur R. Khan Endowed Chair of Structural Engineering and Architecture, and his former Ph.D. student, Alysson Mondoro, integrated for the first time the three most common failure modes for bridges exposed to floods, hurricanes, tsunamis, and other extreme hydrologic events into a comprehensive risk assessment framework.
The work fills a key gap in the way risk for such bridges is assessed over their life-cycle. Their research was published in Engineering Structures (Vol. 159, 2018) in an article titled “Risk-based cost-benefit analysis for the retrofit of bridges exposed to extreme hydrologic events considering multiple failure modes.”
Deck, pier and foundation failure are the three most common bridge failure modes. However, the risk assessment of bridges exposed to hazards have typically included only one or two of these.
“Considering only one or two failure modes provides an incomplete picture because the risk level of each mode differs and, when assessed together, they compete with each other,” says Frangopol. “Our analysis finds that any risk assessment must incorporate all pertinent failure modes of a structure.”
Frangopol and Mondoro illustrate their analytical method using a riverine bridge as an example. They calculated the impact of bridge retrofit actions on possible failure modes in terms of probability of failure, risk, and benefit-cost ratio using a logic modeling technique called an event tree.
In the riverine bridge case study, all of the bridge retrofit options resulted in a reduction in the probability of failure for the examined bridge. However, these options did not provide a unilateral decrease in risk.
For example, the addition of retrofit measures to prevent deck dislodgement decreased the probability of failure of the deck, and, in turn, the bridge. However, it increased the probability of failure of the foundation. Since the consequence of a foundation failure is larger, the overall risk is increased.
“Effective management strategies will vary depending on the bridge and the intensity and frequency of the hazard to which it is exposed,” says Frangopol. “This may be of particular interest in regions where the impacts of natural and anthropogenic climate changes are felt most acutely.”
While the illustrative example focuses on the flooding hazard, the methodology can be applied to other extreme hydrologic events, such as hurricanes and tsunamis.
In another paper on “Adaptation Optimization of Residential Buildings under Hurricane Threat Considering Climate Change in a Life-cycle Context,” recently published in the ASCE Journal of Performance of Constructed Facilities (Vol. 31, No. 6, 2017), Frangopol and his former Ph.D. student You Dong, assistant professor of structural engineering at The Hong Kong Polytechnic University, propose a framework to aid the optimal adaptation of residential buildings considering climate change effects in a life-cycle context. Life-cycle engineering, of which Frangopol is a recognized pioneer, is an approach to assess the environmental impacts in conjunction with economic impacts that includes a structure's life-cycle from its construction to its use and its end. The framework considers the probabilistic occurrence models of hurricanes, structural vulnerability of typical residential buildings, possible climate change scenarios, and optimization of various climate adaptation strategies in a lifecycle context. They applied their approach to a real-world case study: a group of single-family residential buildings located in Miami-Dade County, Florida.
Frangopol’s research on bridge retrofitting is part of an initiative spearheaded by the American Society of Civil Engineers (ASCE). Frangopol, as a member of the ASCE Industry Leaders Council, helps lead ASCE’s efforts to achieve a “Grand Challenge” of reducing the life-cycle cost of U.S. infrastructure by 50 percent by 2025.
“Ultimately, life-cycle engineering is about more than us,” he says. “We’re trying to optimize our world for future generations.”
Developing Resilient Infrastructure
Disaster, either natural or man-made, inevitably strikes at some point, and the recovery of an affected community’s infrastructure systems determines its socio-economic recovery. Another team of Lehigh researchers uses a probabilistic approach to examine how interdependent systems work together during and after a disaster.
This interdisciplinary team, composed by civil engineers, systems engineers, computer scientists, social scientists and economists, is led by Paolo Bocchini, the Frank Hook Assistant Professor in the Department of Civil and Environmental Engineering, and includes Lehigh researchers Snyder; Sause; Lamadrid; and Brian Davison, associate professor of computer science and engineering.
The team focuses on the regional scale rather than the structural scale: How does an entire region recover from disaster?
“Engineers have focused on designing buildings and infrastructure systems able to preserve lives even under extreme events, and they have been quite successful,” Bocchini says. “But in recent years the bar was raised, our society not only asks the built environment to protect lives, but also to remain functional. Even after extreme events, we need our hospitals to be operational, our bridges to be safe, power and water in our houses, and functioning communication systems. Our project aims at modeling, in a probabilistic sense, this complex recovery phase and the interdependencies among the systems involved. We start from the damage in every small structural component, and we zoom out to capture the recovery dynamics of the entire region. With good predictive models, we will be able to optimize mitigation and preparedness efforts.”
Through a $2.2 million NSF grant titled “Probabilistic Resilience Assessment of Interdependent Systems (PRAISys)”—led by Lehigh and with collaborators at Florida Atlantic University and Georgia State University—the team works to “establish and demonstrate a comprehensive framework that combines models of individual infrastructure systems with models of their interdependencies for the assessment the disaster resilience of a community, considering the uncertainty involved.”
“Resilience is a lot more than just thinking about the hard, physical systems, the infrastructure systems,” says Sause. “There are social and economic systems that also get damaged and recover. It’s broader than even engineers tend to think about it, but it’s really about the recovery part of it: planning for it, measuring it, optimizing it.”
Snyder says: “Much of the modeling and research efforts to date had to make strong simplifications and assumptions on the recovery process. For instance, for a given event, they assumed a predefined recovery time for each infrastructure system. In this project, we look at this process in detail, and we account for the randomness in it.”
Tackling Key Challenges
With infrastructure and energy systems underpinning all aspects of modern society, Lehigh’s diverse research community is tackling this work from a variety of angles, within and across disciplines, creating new knowledge and solutions for the challenges facing our most critical systems.
“At Lehigh, I may collaborate with people who are very similar to me—they kind of do what I do, and we speak the same language—but I can just as smoothly collaborate with experts different than me,” says Kishore. “We’re able to look at one problem and use our skill sets to solve the problem together. Then we use that experience to solve an interrelated problem between infrastructure systems. We build on the strengths of one to solve the problems in the second.”
Written by Lori Friedman and Kelly Hochbein
Illustration by Ryan Peltier
Posted on: